The various phospholipid aggregates
Phospholipids can assume different aggregations. Read more about the different phospholipid aggregates.
What aggregates form phospholipids in aqueous dispersion?
The tendency of phospholipids to form ‘superstructures’ in aqueous dispersion is governed by their amphiphilic properties resulting in distinct interaction with the physiological environment. The driving force leading to self-aggregation of phospholipids is the hydrophobic effect originating from the long alkyl chains.1) Since the hydrocarbon chains cannot interact with the surrounding water, the adjacent water molecules are restricted in their movement and are therefore more highly ordered. By aggregating the nonpolar molecular parts of the amphiphiles (phospholipids), the surface area of the molecular parts facing the water is reduced and the number of ordered water molecules is decreased, finally increasing the entropy of the system.
The packing parameter by Israelachvili
The type of aggregates formed depends primarily on the structure of the amphiphiles. Thus, for monopolar, amphiphilic molecules such as phospholipids, it is possible to estimate the expected type of structure via a so-called packing parameter (P) or form factor. P can be calculated from the area of the head group (A), the length of the alkyl chain (lc) and its volume (V).2) Depending on P, the structures formed range from simple spherical and worm-like micelles, to vesicles and planar lipid bilayers, to inverted micelles (see Figure 1). One can further distinguish between lamellar and non-lamellar (hexagonal) phases. It is important to note, however, that the shape of the aggregates is not exclusively determined by the molecular structure. The concentration of the amphiphile, the temperature of the dispersion, and the ionic strength and pH of the solution also play a major role.
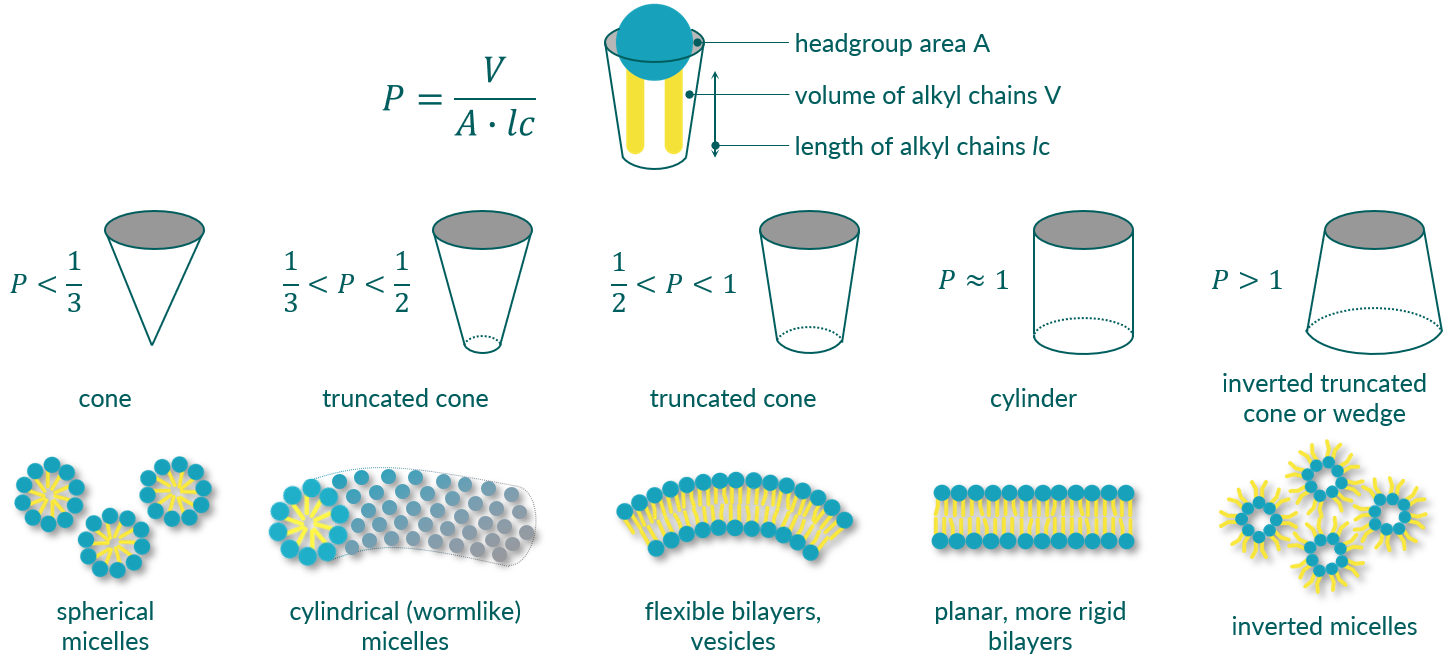
Figure 1. Schematic representation of the different phospholipid aggregates formed in aqueous dispersion in dependence of amphiphile's packing parameter.
Micelles
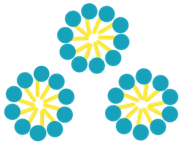
Small spherical micelles are formed in aqueous dispersion by amphiphiles with a relatively small lipophilic fraction. The packing parameter P is less than 1/3. In the case of phospholipids, this can be caused by the presence of only one alkyl chain (lyso-phospholipids) or two short alkyl chains. The hydrodynamic diameter of such micelles is typically in the range of 5 to 10 nm.
Critical micelle concentration (CMC)
Micelles are only formed above a defined concentration of the amphiphile. This concentration is termed Critical Micelle Concentration (CMC). Below this concentration, the amphiphiles are present as single molecules. Of course, one must keep in mind that even above the CMC there is a constant exchange between free amphiphile molecules and amphiphiles bound in micelles.
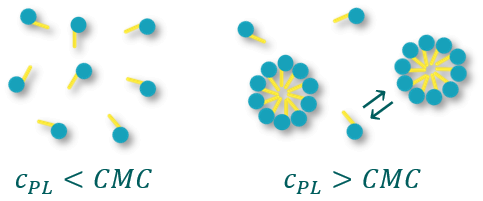
Figure 2. Dependence of micelle formation on phospholipid concentration (cPL)
The CMC is an important property of phospholipids and surfactants in general. Below the CMC, the surface tension changes with the concentration of the amphiphile. Above the CMC, the surface tension remains relatively constant or changes with a smaller slope. The value of the CMC for a given amphiphile depends on the temperature of the medium and the presence and concentration of other surface-active substances and electrolytes.
Cylindrical micelles
If the packing parameter of the amphiphiles is just a tiny bit larger, in the range between 1/3 and 1/2, then elongated, flexible aggregates form in aqueous dispersion. These aggregates can be described as cylindrical, worm-like micelles, and this phase can also be ascribed as hexagonal I (HI) phase. The entanglement to form a network imparts remarkable properties reminiscent of polymer solutions or gels.3) However, these cylindrical micelles can break and reform on a very short timescale. They are also capable of changing their morphology and forming spheres or even vesicles. External parameters such as temperature and pH of the medium can trigger this morphology change.
Lamellar phases, vesicles, and liposomes
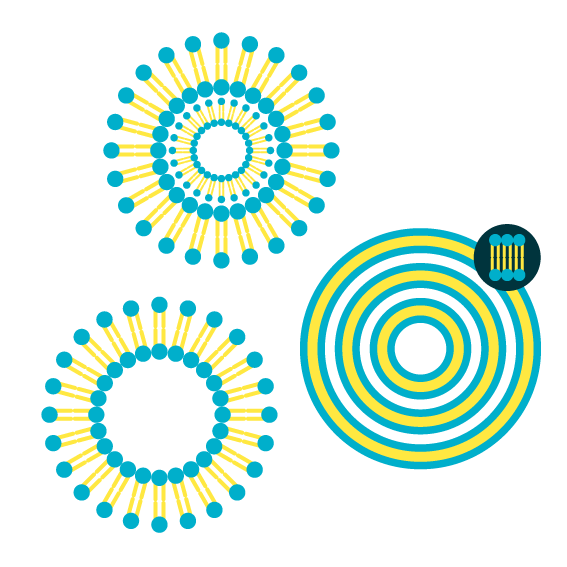
Lamellar phases or phospholipid bilayers are abundantly found in nature, e.g., in cell membranes, and integral part of well-established drug delivery systems like liposomes. Exposed to an aqueous medium, amphiphilic phospholipids assemble into a two-layered sheet with the hydrophobic tails pointing to the inner part and the polar heads to the outer part, resulting in a phospholipid bilayer. This self-assembly occurs when the size, i.e. the cross-sectional area, of the phospholipid's headgroup is approximately equal to the cross-section of its two alkyl chains.
The packing parameter P is between 0.5 and 1. This is the case for nearly all diacyl phosphatidylcholines (PC) and phosphatidylethanolamines (PE). To further minimize the hydrophobic region exposed to water, closed phospholipid vesicles can be formed. These vesicles are also termed liposomes. What makes liposomes so interesting for pharmaceutical applications is the fact that hydrophilic drugs can be encapsulated in the aqueous core of the vesicles. In addition, lipophilic active ingredients can also be "hidden" within the phospholipid layer.
Lamellar repeat distance
The lamellar phase is characterized by repeating phospholipid bilayers separated from each other by an interlamellar water layer (see Figure 3). A typical property of a lamellar phase is the repeat distance (d). This value includes the thickness of the phospholipid bilayer plus the thickness of the water layer. The repeat distance of lamellar phases can be easily determined using small angle X-ray scattering (SAXS) measurments. Many factors can influence the value of the repeat distance: the type of phospholipid (saturated versus unsaturated, length of alkyl chain), the temperature and thus the phase state of the phospholipid and the degree of headgroup hydration, but also the composition of the aqueous phase (ionic strength, type of counterions, pH value).
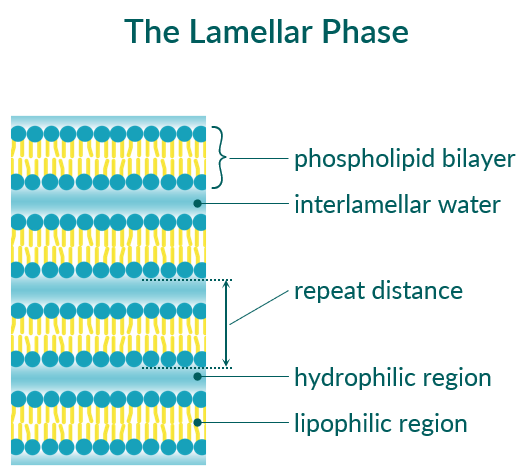
Figure 3. Schematic representation of the lamellar phase of phospholipids.
Types of liposomes
Closed phospholipid bilayers are called vesicles or liposomes. Depending on how many bilayer/vesicles are incorporated in one liposome, one can distinguish between unilamellar vesicles, multilamellar vesicles (MLV), and multivesicular vesicles (MVV, see Figure 4). In the case of liposomes from a single phospholipid bilayer, one can further divide according to their size into:
- small unilamellar vesicles (SUV) with a mean diameter of 20 to 100 nm,
- large unilamellar vesicles (LUV) with a mean diameter of 100 to 1000 nm, and
- giant unilamellar vesicles (GUV) with a mean diameter of 1 to 25 µm or even larger.
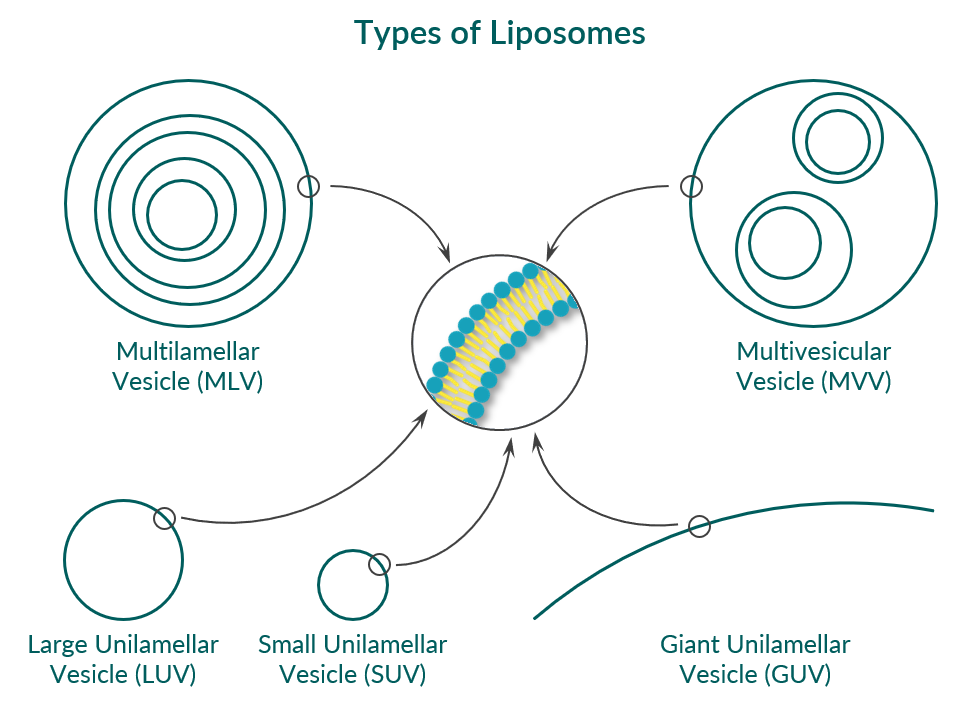
Figure 4. Schematic representation of different types of phospholipid vesicles (liposomes). Cave: the figure is not to scale.
Characterization of liposomes
The most important characteristics of liposomes are their mean diameter and the polydispersity index (PdI), which is a measure of the size distribution. Both can be quantified by dynamic light scattering (DLS) or photon correlation spectroscopy (PCS). The zeta potential, which is a measure of the surface charge of the vesicles, is another typical characteristic of liposomes. The appearance of vesicles can be studied by various electron microscopic (EM) methods. For example, cryo-EM can be used to determine the lamellarity of vesicles, i.e. the number of phospholipid bilayers of a liposome. EM of freeze-fractured replica can elucidate the surface of vesicles. The larger liposomes (GUVs) can also be seen in visible light with an optical microscope.
Production of liposomes
There are various methods for the production of liposomes. The most common are:
- the film method including solvent evaporation and re-hydration with water or buffer,
- the ethanol injection method,
- extrusion through polycarbonate membranes with different diameters,
- sonication, or
- microfluidics.
All these manufacturing methods have specific advantages and disadvantages. Additionally, precautions can be taken to prevent liposomes from fusing. These are, for example, the incorporation of negatively charged phospholipids, such as phosphatidylglycerol (PG) and phosphatic acid (PA) inducing electrostatic repulsion, or lipids with polyethyleneglycol (PEG) chains in the headgroup.
Use of liposomes
Phospholipid vesicles (liposomes) are widely used in pharmaceutical, cosmetic, and dietary applications. Phospholipids are already included in numerous approved drug products, but their potential is far from exhausted. This is due to the very simple reason that phospholipids are extremly well tolerated and non-toxic. Learn more about the use of liposomes and other pharmaceutical formulations including phospholipids on our website.
Hexagonal II phase
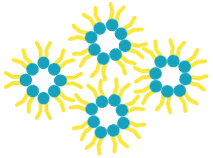
If the packing parameter P of amphiphiles is greater than 1, other non-lamellar phases can be formed, for example, inverted micelles (Hexagonal II phase, HII phase). The packing of phospholipid molecules in the HII phase behaves inversely to that observed in the hexagonal I phase (cylindrical, worm-like micelles). In this phase, the polar phospholipid headgroups are on the inside and the lipophilic tails are on the outside, resulting in the formation of aqueous channels that traverse the entire volume.
Among the most common lipids forming this phase is phosphatidylethanolamine (PE) when it has unsaturated hydrocarbon chains.
Techniques for detection
Various techniques are used to distinguish between the different phases that can form phospholipids depending on phospholipid' concentration, temperature and pH of the solvent.
- Phosphorus nuclear magnetic resonance (31P NMR), different and unique powder diffraction patterns are observed for micellar (isotropic), lamellar, and hexagonal phases
- Small and wide-angle X-ray scattering (SAXS, WAXS)
- Transmission electron microscopy (TEM) of freeze-fractured replica, which offer evidence of the existence of lamellar or hexagonal phases, and of negatively stained samples using uranyl acetate or tungstic acid as contrast agent
- Differential scanning calorimetry (DSC)
You want to know more?
Please use the following links:
What types of phospholipids are there?
What is the difference between natural and synthetic phospholipids?
What is the occurrence and phospholipids benefit?
Which pharmaceutical formulations can be made?
Chapter 1: Wormlike Micelles: An Introduction
in Wormlike Micelles: Advances in Systems, Characterisation and Applications, 2017, pp. 1-8
Google Scholar |